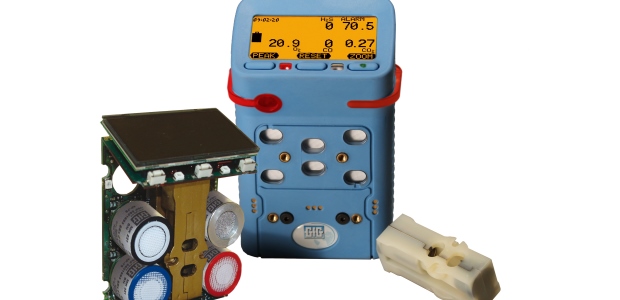
Infrared Sensors Shine a New Light on LEL Combustible Gas Measurement
It is critically important for instrument users to understand what the sensors in their instrument cannot properly measure, as well as what they can.
- By Bob Henderson
- Feb 01, 2016
Non-dispersive infrared (NDIR) sensors are increasingly being used in multi-sensor instruments to measure LEL combustible gas. While they offer a number of significant advantages, NDIR sensors also have very important limitations. No one single sensor (or type of sensor) is capable of detecting all types of dangerous gases and vapors. This is why manufacturers offer several different types of sensors to measure combustible gas and VOC vapors. It's very important to make sure that the measurement technology used in your instrument is the best choice given the nature of the gases you need to measure.
Non-dispersive infrared sensors measure gas as a function of the absorbance of infrared light at a specific wavelength or range of wavelengths. Molecules consist of atoms that are held together by bonds that can vibrate (stretch, bend, or rotate) in three dimensions. When a chemical bond absorbs infrared radiation, the bond continues to vibrate at the same frequency but with greater amplitude after the transfer of energy. For infrared energy to be absorbed (that is, for vibrational energy to be transferred to the molecule), the frequency must match the frequency of the mode of vibration.
When infrared radiation passes through a sensing chamber that contains a measurable gas, only those wavelengths that match the vibration modes of the chemical bonds in the molecules of gas are absorbed. The rest of the light is transmitted through the chamber without hindrance. The instrument measures the amount of light that is absorbed by the molecules in the sensing chamber.
The strength of the NDIR sensor signal is determined by the Beer-Lambert Law, one of the basic laws of physics. Without going into the math, according to Beer's Law, the strength of the signal is proportional to: (1) the intensity of the beam of infrared light, (2) the specific absorbance characteristics of the molecules of gas in the optical sensing chamber, (3) the distance the light travels through the sensing chamber (the optical path length), and of course, (4) the actual concentration of the gas being measured.
For an instrument manufacturer, optimizing performance along one parameter usually requires a tradeoff in performance along another parameter. For instance, using a higher power IR source that produces a brighter beam of light increases signal strength but takes more power. On the other hand, lengthening the optical path length provides greater opportunity for the molecules to absorb the light, improving the sensor's ability to measure gases with lower relative responses, while at the same time allowing use of a lower-power IR source to achieve the same strength of signal. However, it also means that the sensor is physically larger.
As a consequence of these design considerations, the performance characteristics of NDIR sensors can differ significantly between manufacturers. However, all NDIR sensors share certain performance characteristics.
What is the Best Wavelength for Measurement?
The wavelength ranges most frequently used for combustible gas measurement are near 3.33 μm (micrometers) or 3.4 μm. A filter is used to limit the band of wavelengths that are used for measurement. The active detector measures the amount of infrared light absorbed at this wavelength range. A reference detector measures the amount of light at another wavelength where there is no absorbance.
Methane and molecules with double bonds (such as butadiene and ethene) or a benzene ring (such as toluene or benzene) show stronger absorbance nearer 3.33 μm than they do at 3.4 μm, while saturated hydrocarbons such as propane and butane, as well as alcohols, show slightly stronger absorbance at 3.4 μm.
While catalytic Wheatstone bridge type LEL sensors are more sensitive to small molecules like methane than to larger molecules like pentane or hexane, the opposite is true for NDIR sensors. It is the chemical bonds in the molecules being measured that actually absorb the infrared light. Because larger molecules have more chemical bonds holding the atoms in the molecule together, they provide more opportunities for infrared radiation to be absorbed. Of course, the chemical bonds present within the molecule need to absorb IR at the wavelengths being measured.
NDIR sensors cannot measure a gas unless the bonds in the molecules absorb IR at the measurement wavelengths. It is primarily the "stretch" vibrational modes of the C-H bonds in combustible gas molecules that absorb infrared light near 3.33 μm and 3.4 μm. Methane (CH4) has only four C-H bonds. Pentane (C5H12) has 12 C-H bonds. It makes sense that the relative response of the NDIR sensor to pentane is greater than the response to methane.
Any chemical groups in the molecule that depress or reduce the "stretch" vibrational mode reduce the overall absorbance of IR light at these wavelengths. For instance, ethane (C2H6) and ethene (C2H4) are very similar molecules. However, ethene has two fewer hydrogens and includes a C=C double bond that reduces the stretch vibrational mode. Although ethene is still detectable, it has a lower relative response.
Although acetylene (C2H2) has two C-H bonds, the presence of a triple bond between the two carbon atoms so reduces absorbance that it renders the molecule unmeasurable at 3.33 μm or 3.4 μm. However, the C≡C triple bond shows strong absorbance at a wavelength of 3.01 μm. Unfortunately, while acetylene absorbs strongly at 3.01 μm, most other commonly encountered combustible gases do not, making the wavelength a poor choice for general LEL measurement.
Instruments equipped with NDIR sensors for combustible gas measurement generally include a library of response curves. For methane or natural gas measurement, it is generally best to use the methane scale and calibrate the sensor using methane gas. For measurement of alcohols and saturated hydrocarbons (such as ethane, propane, butane, pentane, hexane, nonane, etc.), it is generally better to operate the instrument on the propane scale, because the relative responses of these gases and vapors are fairly similar to each other. For unsaturated gases such as ethylene or benzene, direct calibration to the gas of interest is normally the best approach.
Limitations of Catalytic Wheatstone Bridge Type LEL Sensors
NDIR combustible gas sensors have a number of advantages when compared to traditional Wheatstone bridge type sensors. Wheatstone bridge type LEL sensors include a flame arrestor that can slow larger hydrocarbon molecules from entering the sensor. The larger the molecule, the slower it diffuses through the flame arrestor into the heated bead (pellistor) in the sensor where it is oxidized. Small combustible gas molecules such as hydrogen (H2), methane (CH4), and propane (C3H8) diffuse rapidly into the bead and produce a strong relative response. Larger molecules such as hexane (C6H14) and octane (C8H18) take longer to reach and penetrate the active bead in the sensor and have a much lower relative response.
Saturated hydrocarbons larger than nonane (C9H20) take so long to reach and penetrate the active bead that they are essentially unmeasurable by standard LEL sensors. To put this in perspective, less than 4 percent of the molecules in a bucket of diesel fuel are small enough to be measurable by means of a traditional LEL sensor. This is one of the reasons that Wheatstone bridge type LEL sensors show such a low response when exposed to the vapors of "heavy" fuels such as diesel, kerosene, jet fuel, and heating oil.
Advantages of NDIR LEL Sensors
- Intrinsically safe NDIR sensors do not need to be equipped with external flame arrestors that can slow diffusion into the sensor and do not depend on gas penetrating the bead in order to be oxidized and detected. Larger molecules, such as the saturated aliphatic molecules in heavy fuel vapor, absorb more IR light and produce a stronger signal than smaller molecules, such as methane and propane.
- NDIR sensors do not require oxygen.
- NDIR sensors are not damaged by exposure to traditional LEL sensor poisons such as volatile silicones, high concentration sulfides, hydrides like phosphine and arsine, and halogenated solvents and degreasers, such as methylene chloride.
- NDIR sensors can be used for measurement of high concentration gas up to 100 percent volume.
Limitations of NDIR Sensors
NDIR sensors cannot be used to measure gases that do not absorb infrared light. "Diatomic" molecules, such as oxygen (O2), nitrogen (N2), and hydrogen (H2), do not absorb infrared light. In applications where H2 may be potentially present, the instrument should be equipped with a sensor designed to respond to H2, such as a traditional type LEL sensor, or an electrochemical sensor capable of directly measuring H2 in the desired range.
NDIR sensors can be used only for gases that absorb IR light at the wavelengths being measured. For instance, NDIR sensors that measure at 3.33 μm or 3.4 μm cannot be used to measure acetylene.
The NDIR sensor must have a strong enough response to measure the gas or gases of interest. For instance, if you need to measure a mixture of gases that includes chemicals with double bonds, or aromatic VOC gases with benzene rings, make sure to talk with the manufacturer about the relative response of the sensor to these gases.
Conclusion
The sensors utilized in portable gas detectors are extremely good at detecting what they are designed to measure. The problem is that users are frequently unaware of the limitations and use the sensors in ways that result in inaccurate readings. It is critically important for instrument users to understand what the sensors in their instrument cannot properly measure, as well as what they can.
This article originally appeared in the February 2016 issue of Occupational Health & Safety.